My Research:
1. Star Formation and Young Star Clusters in the Milky Way and Magellanic
Clouds
Preamble
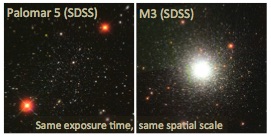
The work presented in this section was done with my past and present graduate students Andrea Dieball, Katharina Glatt, Andrea Kayser, Andreas Koch, Xiaoying Pang, Andrea Stolte, and Peter Zeidler.
Subtopics
- 1.1 The Stellar Initial Mass Function (IMF) and Mass Segregation
- 1.2 Effects of Massive Star Formation on Low-Mass and Sequential Star Formation
- 1.3 Emission-line Stars in Young Clusters
- 1.4 Age Spreads
- 1.5 Binary and Multiple Star Clusters
1.1 The Stellar Initial Mass Function (IMF) and Mass Segregation
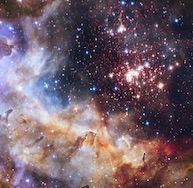
One way of addressing these questions is by directly determining the IMF in very young clusters; clusters young enough to not yet have been significantly affected by dynamical or stellar evolution. Our studies of young massive clusters with ground-based adaptive optics or with HST revealed such empirical measurements of the IMF to be more difficult than expected. My graduate students Andrea Stolte, Xiaoying Pang, and Peter Zeidler and I investigated several young massive clusters in detail (Arches (age 2 - 4 Myr), NGC 3603 (~ 1 Myr), and Westerlund 2 (~ 1 Myr)). They all turned out to show mass segregation despite their young age. We found radially varying mass functions and a concentration of high-mass stars in the cluster centers (e.g., Stolte et al. 2002, 2005; Pang et al. 2013). Interestingly, our results favor dynamical over primordial mass segregation, which may have implications for formation scenarios of very massive stars (Pang et al. 2013). In the Hubble Tarantula Treasury Project (HTTP; Sabbi et al. 2013), we are now extending these studies to the young clusters in 30 Doradus in the Large Magellanic Cloud (LMC).
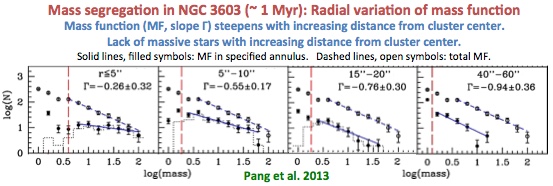
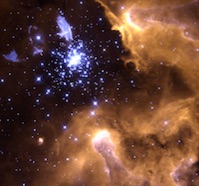
Massive star formation in very dense environments may affect low-mass star formation in its vicinity and/or trigger subsequent star formation activity. In NGC 3603 we found the disk fraction for pre-main-sequence stars to increase from the center to the outskirts, indicating that photoevaporation caused by massive stars shortens the mass accretion time scale (e.g., Stolte et al. 2004). In Westerlund 2 we found the mass accretion rate of the pre-main-sequence stars to be 25% lower in close spatial proximity to main-sequence OB stars (Zeidler et al., in prep.). A young cluster's ionizing radiation also has a substantial impact on the surrounding nebula, clearing a cavity around the cluster and eroding the remaining pillars with higher gas and dust density as well as the envelopes of proplyds (e.g., Brandner et al. 2000; Pang, Pasquali, & Grebel 2011).
In very massive star-forming regions like 30 Doradus star clusters with a range of ages can be found (e.g., Grebel & Chu 2000). Here, we discovered the first pre-main-sequence stars detected in a galaxy other than the Milky Way and found evidence of sequential star formation presumably triggered by the central massive R136 cluster (Walborn et al. 1999; Brandner et al. 2001). The infrared HST data even revealed a very young, embedded, compact cluster in the vicinity of R136. We are now resuming these studies in the framework of the HTTP imaging survey with HST (e.g., Cignoni et al. 2015; Sabbi et al. 2015).
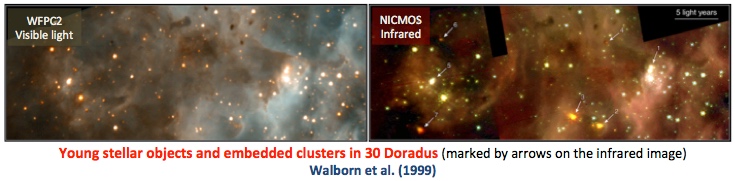
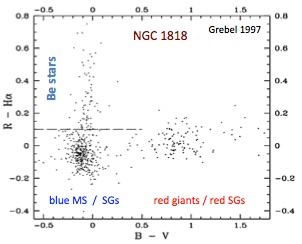
Young star clusters may contain various types of emission-line stars, for instance He I and He II emission-line stars such as O and B supergiants or He II-emitting WN stars, C III-emitting WC stars, or Balmer emission-line stars such as Be stars or pre-main-sequence stars. We developed a simple photometric method for the detection of such stars, which uses a narrow-band filter centered on the desired emission line in combination with broad-band filters to measure the continuum and colors of the stars (Grebel, Richtler, & de Boer 1992).
Applying this method to search for main-sequence Be stars in young clusters, we found that the Be star fraction among B main-sequence stars is highest among early-type B stars (Grebel et al. 1992, 1993; Grebel 1997; Grebel & Chu 2000; Keller et al. 2001). Furthermore, comparing clusters the Milky Way, the Large Magellanic Cloud, and the Small Magellanic Cloud, we found the Be star fractions to increase with lower metallicities (Grebel 1997; Maeder, Grebel, & Mermilliod 1999). We suggested that that the average rotation is faster at low metallicities, which in turn implies more rotational mixing and could explain the higher relative N enrichment found in massive metal-poor stars (Maeder et al. 1999).
Pre-main-sequence stars also show varying amounts of Hα emission depending on their mass accretion rate. In the young Galactic cluster Westerlund 2, we used our photometric technique to identify pre-main-sequence stars, to quantify their mass accretion rate, and to show that circumstellar disks around these low-mass stars get rapidly destroyed by the UV radiation emitted by massive OB stars (Zeidler et al., in prep.)
1.4 Age Spreads
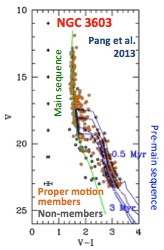
Nonetheless, the currently available data suggest that in young compact massive clusters age spreads, if any, seem to be small. For instance, a study of a careful proper-motion-based sample of member stars in the massive Galactic star cluster NGC 3603 by my graduate student Xiaoying Pang indicates an approximate mean age of 1 Myr for this object. The broad main-sequence turn-on region of the cluster supports an age spread of possibly up to two Myr, and the few older main-sequence member stars with lower luminosities than the bulk of the main sequence also suggest an earlier onset of star formation (Pang et al. 2013). However, it is also evident that star formation already progressed in that region prior to the formation of the massive cluster as evidenced by, e.g., the evolved supergiants found in its vicinity (e.g., Brandner et al. 1997a, 1997b). That young star clusters are embedded in environments with earlier as well as continuing star formation in their surroundings is also observed in many other extended massive star-forming regions, for instance in 30 Doradus (e.g., Grebel & Chu).
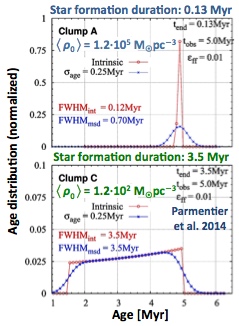
The discovery of multiple stellar populations in globular clusters (see Section 2.1) may indicate the presence of two or more stellar generations of different ages. This has reinvigorated the interest in possible age spreads in star clusters, particularly with respect to populations separated in age. If there are indeed multiple stellar generations, when and how did they form? Our Milky Way does not contain massive intermediate-age clusters in which one could catch such events as they are happening, but the Magellanic Clouds are rich in such clusters.
In a study to age-date intermediate-age clusters in the Small Magellanic Cloud (SMC) led my my graduate student Katharina Glatt, we detected the first cluster with possible multiple main-sequence turn-offs ever to be found in the SMC: NGC 419 (Glatt et al. 2008). Since then a growing number of such Magellanic clusters with ages typically between one to two Myr have been identified, but whether the extended turn-off regions seen in their color-magnitude diagrams are indeed caused by age spreads is a matter of debate. Other explanations, such as stellar rotation, have been proposed as well, which obviate the need for an age spread (e.g., Niederhofer et al. 2015 and references therin). Indeed, somewhat older massive intermediate-age clusters with ages of several Gyr appear to be entirely consistent with being single stellar populations (Glatt et al. 2008).
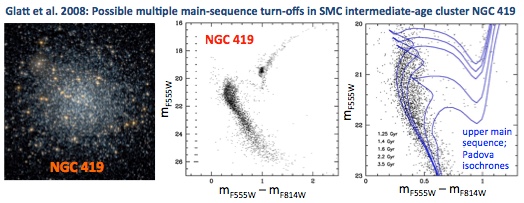
1.5 Binary and Multiple Star Clusters
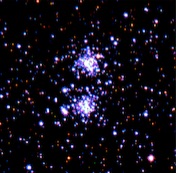
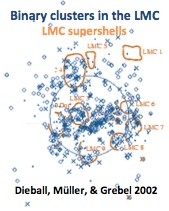
References
- Bhatia, R.K., & Hatzidimitriou, D. 1988, MNRAS, 230, 215
- Blaauw, A. 1991, in The Physics of Star Formation and Early Stellar Evolution, NATO Advanced Science Institutes (ASI) Series C, Vol. 342, eds. C.J. Lada & N.D. Kylafis (Dordrecht: Kluwer), p. 125
- Brandner, W., Grebel, E.K., Chu, Y.-H., & Weis, K. 1997a, ApJL, 475, L45
- Brandner, W., Chu, Y.-H., Eisenhauer, F., Grebel, E.K., & Points, S.D. 1997b, ApJL, 489, L153
- Brandner, W., Grebel, E.K., Chu, Y.-H., et al. 2000, AJ, 119, 292
- Brandner, W., Grebel, E.K., Barbá, R.H., Walborn, N.R., & Moneti, A. 2001, AJ, 122, 858
- Cignoni, M., Sabbi, E., van der Marel, R.P., Tosi, M., Zaritsky, D., Anderson, J., Lennon, D.J., Aloisi, A., de Marchi, G., Gouliermis, D.A., Grebel, E.K., Smith, L.J., & Zeidler, P. 2015, ApJ, 811, 76
- Dieball, A., & Grebel, E.K. 1998, A&A, 339, 773
- Dieball, A., & Grebel, E.K. 2000, A&A, 358, 897
- Dieball, A., Grebel, E.K., & Theis, C. 2000, A&A, 358, 144
- Dieball, A., Müller, H., & Grebel, E.K. 2002, A&A, 391, 547
- Glatt, K., Grebel, E.K., Sabbi, E., Gallagher, J.S., Nota, A., Sirianni, M., Clementini, G., Tosi, M., Harbeck, D., Koch, A., Kayser, A., & Da Costa, G. 2008, AJ, 136, 1703
- Grebel, E.K. 1997, A&A, 317, 448
- Grebel, E.K., & Chu, Y.-H. 2000, AJ, 119, 787
- Grebel, E.K., Richtler, T., & de Boer 1992, A&A, 254, L5
- Grebel, E.K., Roberts, W.J., Will, J.-M., & de Boer, K.S. 1993, SSRv, 66, 65
- Grebel, E.K., Roberts, W.J., & Brandner, W. 1996, A&A, 311, 470
- Keller, S.C., Grebel, E.K., Miller, G.J., & Yoss, K.M. 2001, AJ, 122, 248
- Maeder, A., Grebel, E.K., & Mermilliod, J.-C. 1999, A&A, 346, 459
- Niederhofer, F., Georgy, C., Bastian, N., & Ekström, S. 2015, MNRAS, 453, 2070
- Pang, X., Pasquali, A., & Grebel E.K. 2011, AJ, 142, 132
- Pang, X., Grebel, E.K., Allison, R.J., et al. 2013, ApJ, 764, 73
- Parmentier, G., Pfalzner, S., & Grebel, E.K. 2014, ApJ, 791, 132
- Sabbi, E., Anderson, J., Lennon, D.J., van der Marel, R.P., Aloisi, A., Boyer, M.L., Cignoni, M., De Marchi, G., de Mink, S.E., Evans, C.J., Gallagher, J.S., Gordon, K., Gouliermis, D.A., Grebel, E.K., et al. 2013, AJ, 146, 53
- Sabbi, E., Lennon, D.J., Anderson, J., Cignoni, M., van der Marel, R., Zaritsky, D., de Marchi, G., Panagia, N., Gouliermis, D., Grebel, E.K., et al. 2016, ApJS, 222, 11
- Stolte, A., Grebel, E.K., Brandner, W., & Figer, D.F. 2002, A&A, 394, 459
- Stolte, A., Brandner, W., Brandl, B., Zinnecker, H., & Grebel, E.K. 2004, AJ, 128, 765
- Stolte, A., Brandner, W., Grebel, E.K., Lenzen, R., & Lagrange, A.-M. 2005, ApJ, 628, L113
- Walborn, N.R., Barbá, R.H., Brandner, W., Rubio, M., Grebel, E.K., & Probst, R.G. 1999, AJ, 177, 225
- Zeidler, P., Sabbi, E., Nota, A., Grebel, E.K., Tosi, M., Bonanos, A.Z., Pasquali, A., Christian, C., de Mink, S.E., & Ubeda, L. 2015, AJ, 150, 78
Back to research overview